Lead optimization is an exhausting process of making small changes in biologically active hits to obtain suitable drug candidates for further clinical trials.
One of the fundamental strategies that can be used in Medicinal Chemistry for this purpose is a bioisosteric substitution of some structural motifs or parts of functional groups [1]. Such replacement can lead to better bioavailability and an increase in metabolic stability. Other possible advantages of using bioisosteres are beneficial changes in lipophilicity, solubility, and permeability.
In a recent article by N.A. Meanwell the current state-of-art in the usage of bioisosteres in Drug Discovery was described [2]. Inspired by this review, we have prepared several sets of building blocks that share various fragments mentioned in the article. Carboxylic acids bioisosteres, various fluorine-containing motifs, and some isomers of disubstituted phenyl ring are among them.
We offer a variety of bioisosteres of such groups!
The first set is devoted to bioisosteres of a carboxylic acid moiety which is known to be a fundamental part of many biologically active compounds. Over 450 worldwide marketed drugs contain this group (including antibiotics, anti-coagulants, cholesterol medications, etc.) [3]. However, in some cases, the presence of a carboxylic acid group in a drug candidate can be associated with deficient pharmacokinetic properties and toxicity. The usage of carboxylic acid bioisosteres can be a promising strategy for avoiding these drawbacks. The most prominent carboxylic acid isosteres in marketed drugs are tetrazoles, acyl sulfonamides, hydroxamic acids, 3-hydroxy oxetanes, and several other acyclic and heterocyclic moieties.
Discover Chemspace Carboxylic Acid Bioisosteres – a library of in-stock compounds that contains more than 15 isosteric types and can be used in library design for further investigations.
The second set in bioisosteric replacements is isosteres of a tert-butyl group.
The tert-butyl group is spread among approved medicines and is widely used in drug design [4]. But its presence can lead to undesirable properties such as increased lipophilicity and reduced metabolic stability. Compounds with a fluorinated tert-butyl group, as well as trifluoromethyl derivatives of cyclopropane, cyclobutane, and oxetane, which mimic the bulky proportions and non-polar character of the tert-butyl group, are proposed as its replacements in the drug design process [5,6].
This flexibility in the design process helps to optimize the properties of the lead compounds by improving the potency, bioavailability, and ADME profiles, as evidenced by research in the field of medicinal chemistry.
Discover Chemspace tert-Butyl Group Bioisosteres – a library of 160 in-stock compounds that can mimic the properties of this group and be used in library design for further studies.
The next broad group of bioisosteres that we offer is the metaphors of disubstituted phenyl rings.
The benzene ring is the most spread in commercially available drugs and compounds under clinical trials [2]. Numerous detailed analyses of compound properties have shown that the presence of flat phenyl rings in molecules is a crucial factor contributing to unfavorable properties in molecules facing drug development challenges [7].
These observations have inspired the elaboration of potential bioisosteric replacements for para-, meta-, or ortho- disubstituted benzene rings that could lead to improved molecule lipophilicity and solubility, provide molecules with better ADME profiles, and generate patentable compounds [8].
Discover Chemspace meta-Substituted Benzene Bioisosteres – a library of 260 in-stock compounds that can mimic phenyl ring properties and potentially enhance physicochemical and pharmacokinetic characteristics of molecules in the drug development process.
Over three hundred drugs and agrochemicals contain ortho-substituted benzene rings [9,10]. However, the presence of several phenyl rings in a molecule can lead to undesirable properties, including toxicity. To mitigate such issues, it is proposed to replace the benzene fragment in the molecule, which is not involved in specific ligand-target interactions.
Saturated and conformationally rigid bicyclic molecules, such as 1,2- and 1,5-disubstituted bicyclo[2.1.1]hexanes and 2-oxabicyclo[2.1.1]hexanes, are suggested as bioisosteric replacements for ortho-disubstituted phenyl rings [9-11]. These substitutions represent a promising strategy for obtaining new patentable molecules with improved bioactivity and physicochemical parameters.
Discover Chemspace ortho-Substituted Benzene Bioisosteres – a library of 120 in-stock compounds featuring saturated bicyclic scaffolds that can potentially mimic 1,2-disubstituted phenyl ring properties. Replacement with these moieties represents a promising tactic leading to enhanced water solubility and decreased lipophilicity with retained biological activity.
More than five hundred drugs and agrochemicals comprise the para-substituted phenyl ring as part [12]. However, organic compounds containing more than two benzene rings frequently encounter water solubility challenges. To address these concerns, it was suggested to replace the benzene ring with conformationally rigid, metabolically stable, and non-chirality fragments.
Bicyclo[1.1.1]pentanes, bicyclo[2.2.2]octanes, and cubanes were proposed as the prime saturated bioisosteres of the para-substituted phenyl ring [13-15]. Additionally, 2-oxabicyclo[2.2.2]octane and spiro[3.3]heptane derivatives represent the newly designed, synthesized, and validated saturated bioisosteres with improved physicochemical properties [12,16]. The increase in the range of available bioisosteres of aromatic rings is an encouraging trend in Drug Discovery for the synthesis of refined and patent-free drug candidates.
Discover Chemspace para-Substituted Benzene Bioisosteres – a library of 500 in-stock compounds containing saturated cores that can potentially replace 1,4-disubstituted phenyl ring in drug design. Substituting benzene rings in bioactive compounds with such fragments offers a promising strategy that enhances water solubility and reduces lipophilicity while maintaining biological activity.
You can also find information about Bioisosteric Replacements by downloading a PDF.
For any questions, please contact us at sales@chem-space.com.
1. Meanwell, N. A. (2011). Synopsis of Some Recent Tactical Application of Bioisosteres in Drug Design. Journal of Medicinal Chemistry, 54, 2529–2591. doi:10.1021/jm1013693
2. Meanwell, N. A. (2023). Applications of Bioisosteres in the Design of Biologically Active Compounds. Journal of Agricultural and Food Chemistry. doi:10.1021/acs.jafc.3c00765
3. Bredael, K., Geurs, S., Clarisse, D., De Bosscher, K. & D’hooghe, M. (2022). Carboxylic Acid Bioisosteres in Medicinal Chemistry: Synthesis and Properties. Journal of Chemistry, 2022, 1–21. doi:10.1155/2022/2164558
4. Westphal, M. V., Wolfstädter, B. T., Plancher, J.-M., Gatfield, J. & Carreira, E. M. (2015). Evaluation of tert-Butyl Isosteres: Case Studies of Physicochemical and Pharmacokinetic Properties, Efficacies, and Activities. ChemMedChem, 10, 461–469. doi:10.1002/cmdc.201402502
5. Mukherjee, P., Pettersson, M., Dutra, J. K., Xie, L. & am Ende, C. W. (2017). Trifluoromethyl Oxetanes: Synthesis and Evaluation as a tert-Butyl Isostere. ChemMedChem, 12, 1574–1577. doi:10.1002/cmdc.201700333
6. Kawamura, K., Yoshioka, H., Sato, C., Yajima, T., Furuyama, Y., Kuramochi, K. & Ohgane, K. (2023). Fine-tuning of nitrogen-containing bisphosphonate esters that potently induce degradation of HMG-CoA reductase. Bioorganic & Medicinal Chemistry, 78, 117145. doi:10.1016/j.bmc.2022.117145
7. Subbaiah, M. A. M. & Meanwell, N. A. (2021) Bioisosteres of the Phenyl Ring: Recent Strategic Applications in Lead Optimization and Drug Design. Journal of Medicinal Chemistry, 64, 14046–14128. doi:10.1021/acs.jmedchem.1c01215
8. Frank, N., Nugent, J., Shire, B. R., Pickford, H. D., Rabe, P., Sterling, A. J., Zarganes-Tzitzikas, T., Grimes, T., Thompson, A. L., Smith, R. C., Schofield, C. J., Brennan, P. E., Duarte, F. & Anderson, E. A. (2022) Synthesis of meta-substituted arene bioisosteres from [3.1.1]propellane. Nature 611, 721–726. doi:10.1038/s41586-022-05290-z
9. Denisenko, A., Garbuz, P., Shishkina, S. V., Voloshchuk, N. M. & Mykhailiuk, P. K. (2020). Saturated Bioisosteres of ortho‐Substituted Benzenes. Angewandte Chemie International Edition, 59, 20515–20521. doi:10.1002/anie.202004183
10. Denisenko, A., Garbuz, P., Voloshchuk, N. M., Holota, Y., Al-Maali, G., Borysko, P. & Mykhailiuk, P. K. (2023). 2-Oxabicyclo[2.1.1]hexanes as saturated bioisosteres of the ortho-substituted phenyl ring. Nature Chemistry, 15, 1155–1163. doi:10.1038/s41557-023-01222-0
11. Denisenko, A., Garbuz, P., Makovetska, Y., Shablykin, O., Lesyk, D., Al-Maali, G., Korzh, R., Sadkova, I. & Mykhailiuk, P. (2023). 1,2-Disubstituted Bicyclo[2.1.1]hexanes as Bioisosteres of the ortho-substituted Benzene. ChemRxiv. doi:10.26434/chemrxiv-2023-78z7p
12. Levterov, V. V., Panasiuk, Y., Sahun, K., Stashkevych, O., Badlo, V., Shablykin, O., Sadkova, I., Bortnichuk, L., Klymenko-Ulianov, O., Holota, Y., Lachmann, L., Borysko, P., Horbatok, K., Bodenchuk, I., Bas, Y., Dudenko, D. & Mykhailiuk, P. K. (2023). 2-Oxabicyclo[2.2.2]octane as a new bioisostere of the phenyl ring. Nature Communications, 14, 5608. doi:10.1038/s41467-023-41298-3
13. Stepan, A. F. et al. (2012). Application of the Bicyclo[1.1.1]pentane Motif as a Nonclassical Phenyl Ring Bioisostere in the Design of a Potent and Orally Active γ-Secretase Inhibitor. Journal of Medicinal Chemistry, 55, 3414–3424. doi:10.1021/jm300094u
14. Chalmers, B. A. et al. (2016). Validating Eaton’s Hypothesis: Cubane as a Benzene Bioisostere. Angew Chem Int Ed 55, 3580–3585. doi:10.1002/anie.201510675
15. Auberson, Y. P., Brocklehurst, C., Furegati, M., Fessard, T. C., Koch, G., Decker, A., La Vecchia, L. & Briard, E. (2017). Improving Nonspecific Binding and Solubility: Bicycloalkyl Groups and Cubanes as para‐Phenyl Bioisosteres. ChemMedChem 12, 590–598. doi:10.1002/cmdc.201700082
16. Prysiazhniuk, K., Datsenko, O., Polishchuk, O., Shulha, S., Shablykin, O., Nikandrova, L., Horbatok, K., Bodenchuk, I., Borysko, P., Shepilov, D., Pishel, I., Kubyshkin, V. & Mykhailiuk, P. (2023). Spiro[3.3]heptane as a Non-collinear Benzene Bioisostere. ChemRxiv, doi:10.26434/chemrxiv-2023-djc05
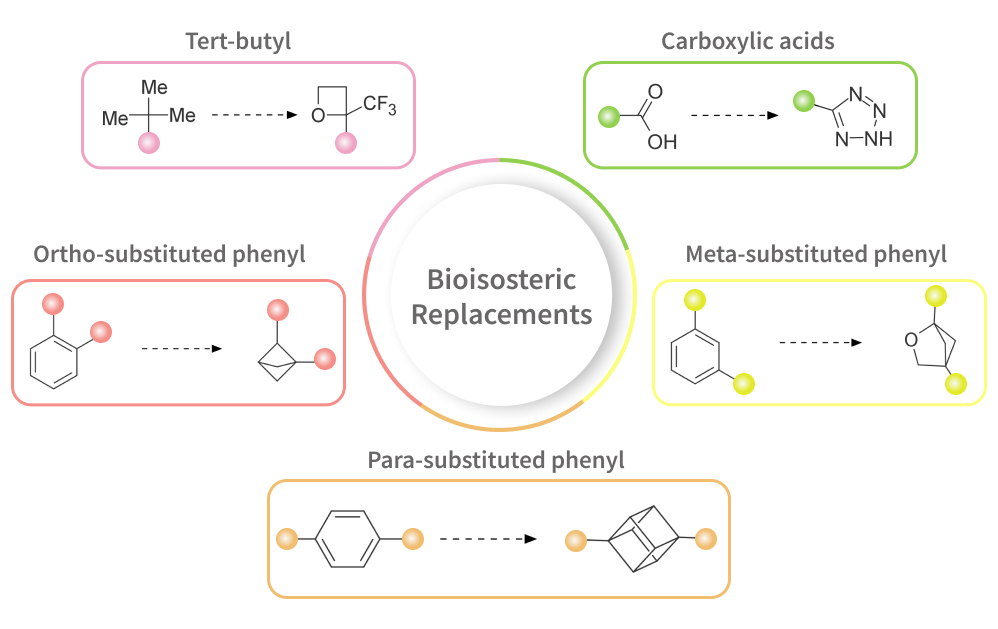